Dynamics and energetics of a sphere during water exit
By Yang Huang, Professor Qing Xiao
By using a multi-phase Navier–Stokes solver combining the volume of fluid approach for water–air interface, the large-eddy simulations method for turbulence effect, and the overset mesh technique for moving boundary, we investigate the dynamics and energetics of a neutrally buoyant sphere during water exit process. The sphere is launched vertically with various initial velocities. In our analysis, the water exit process is divided into three distinct phases, fully submerged, partially submerged, and airborne. The focus is on the roles of the gravity, buoyancy, viscous, and wave-radiation forces in determining the motion and energy exchange/dissipation. During the fully submerged phase, the energy loss of the sphere is caused by the viscous force and the wave-radiation force, with the former playing the dominating role. In the partially submerged phase, the buoyancy force decreases as the submerged volume is reduced. However, under certain conditions, there could be an additional supporting force on the sphere caused by upward water flow beneath it. Once the sphere is fully airborne, its motion is primarily governed by gravity, and the maximum height it attains correlates strongly with the water-exit speed. These findings offer deeper insights into optimizing underwater launch parameters and understanding energy transfer mechanisms in water exit scenarios.
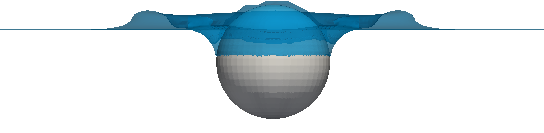
Modular Magnetic Bio-Inspired Autonomous Underwater Vehicle
By Marvin Wright, Professor Qing Xiao
The novel robotic system promises increased swimming efficiency and manoeuvrability by adopting fish swimming motion, which will enable it to operate for longer and in more complex environments. With a target application of underwater inspection of marine assets such as offshore renewable energy devices, the small size untethered system provides access to tight underwater spaces and aims for continuous deployment reducing time to action and avoiding costly human operation and deployment cost. The highly flexible modular system achieves further cost reduction through common module architecture and enables flexible system setup general payload and sensor equipment.
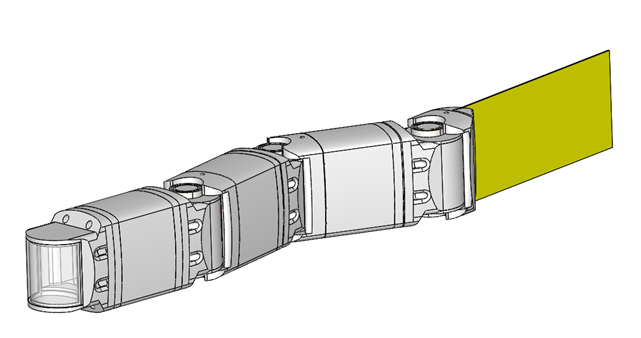
Hydrodynamics of soft-body bio-inspired underwater vehicle, biomimetic underwater propulsion, and analysis of stability of bio-inspired robotic fish using CFD
By Yang Luo, Professor Qing Xiao
Natural selection, survival of the fittest. Marine organisms have many features suitable for the survival of underwater environment, and they can achieve extraordinary propulsion efficiencies, acceleration and maneuverability with low noise. Therefore, it is of great significance to study bio-inspired propulsion to develop underwater vehicle with high performance.
The current research indicate that deformable/flexible fins have better propulsion performance than rigid counterparts. However, the underlying mechanism still remains not so clear to us. Our research group have been focusing on the principle behind it using advanced computational fluid dynamics (CFD) techniques. And some exciting findings have been brought by us which can be found in our previous publications.
My current research aims at further developing our in-house code by extending the in-built structural model. Through solving the coupled fluid and structural governing equations, we can investigate the interaction of flow and the soft fins and body of fishes in swimming. Thus, it will help us to have a better understanding of the propulsion in marine and provide a reference for the future design of underwater vehicle.
CFD and control coupling for numerical-simulations-on-bio-inspired-swimming-robots
By Marvin Wright, Professor Qing Xiao
Numerical simulations are conducted in ANSYS Fluent 19.2 coupled with an in-house developed mobile multibody dynamics and control User Defined Function (UDF). The UDF enables the simulation of the internal and external dynamics and body control of an unfixed multi body system connected by revolving joints and its FSI induced 6DoF (3DoF for 2D case) motion in the inertia frame. At equilibrium position the NACA0012 shaped swimmer is divided into 10 elements (9 joints) with no separating gap. Via prescribed sinusoidal rotation of the joints, with an amplitude and phase distribution along the whole body, the swimmer mimics BCF carangiform fish swimming motion. Furthermore, turning in 2 dimensional plane (Yaw) is achieved by adding camber along the body. The presented motion of the swimmer is following velocity and yaw set points achieved by two separate PID controller. The below video shows a fully PID controlled U turn manoeuvre from an initial still body.
A fluid-structure interaction study on a passively deformed fish fin
By Yang Luo, Professor Qing Xiao
In this project, the propulsive performance of a caudal peduncle-fin swimmer mimicking a bio-inspired robotic fish model is numerically studied using a fully coupled FSI solver. The model consists of a rigid peduncle and a flexible fin which pitches in a uniform flow. The flexible fin is modelled as a thin plate assigned with non-uniformly distributed stiffness. A finite volume method based in-house Navier-Stokes solver is used to solve the fluid equations while the fin deformation is resolved using a finite element code. The effect of the fin flexibility on the propulsive performance is investigated.
The numerical results indicate that compliance has a significant influence on propulsive performance. Under the parameters studied in this research, the medium flexible fin exhibits remarkable efficiency improvement, as well as the thrust augment, while the least flexible fin shows no obvious difference from the rigid one. However, for the most flexible fin, although the thrust production decreases sharply, the efficiency reaches the maximum value. Interestingly, by non-uniformly distributing the rigidity across the caudal fin, our model is able to replicate some fin deformation patterns observed in both the live fish and the experimental robotic fish.
Understanding Bio-Inspired Robot Propulsion, Maneuvering, and Power Hydrodynamic Control and Autonomous Docking Study
By Marvin Stuart Wright, Professor Qing Xiao, Dr Mark Post
Autonomous underwater vehicles (AUV) revolutionized scientific and industrial monitoring, investigation and exploration of the oceans. The highly anticipated characteristics and recent technology advances are a driving force for further research and development in this area. However, up until now there are still various limitations to the fully autonomous and economically successful application of such systems.
The research will focus on reducing such limitations, especially in terms of autonomy to further enable long term self-sufficient deployment without human intervention. In order to achieve an extended self-sufficient period, the AUV’s ability to dock for recharging and information transfer is vital. Such docking activity requires high manoeuvrability, precision and robustness in control and system design. To fully understand the systems functionality within the ocean environment a hydrodynamic performance analysis and control and system design study will be carried out.
With emphasis on enhancing the overall efficiency of an AUV system, special focus will be put on biomimetic concepts and technologies.
Multi-body Dynamics Modelling on a Self-propelled Pufferfish with Rigid Fins
By Ruoxin Li, Professor Qing Xiao
In the present research, a self-propelled pufferfish with three rigid fins, i.e. caudal, dorsal and anal fins, is modelled with our in-house multi-body dynamics code. The model is extracted from a live fish experiment, which was tested at Shanghai Jiao Tong University (SJTU), China. The motion of the fish fins is also obtained from the experiment and the locomotion of the fish body is fully induced by the oscillating motion of fish fins and entirely determined by computation. Hydrodynamic forces are integrated using a Computational Fluid Dynamics method in the commercial software package Fluent.
The following animation shows the self-propelled fish motion in still water as well as the vorticity contour coloured by pressure. In this case, the phase angles of dorsal and anal fins are identical while there is a phase angle difference of pi for the caudal fin.
Pharmaceutical Influences on Locomotion of Acquatic Animals by Experimental Measurements and Numerical Simulations
By Zhenkai Zhao, Professor Qing Xiao
There are two types of muscles in most of the animals including zebra fish, one is called white muscle, which is responsible for the acceleration; another one is called red muscle that is responsible for sustainable low speed. As can be seen in Figure 1, muscle dystrophy will lead to the detachment of muscle fiber that is related to the swimming velocity of zebra fish. Besides, the change of muscle connections will have an influence on the energy transmission to the tail and causes different propulsion efficiency as well as other related parameters. Thus, in order to have a better understanding of the distribution of muscle dystrophy, a novel idea of analyzing forces and moments based on experimental measurements and numerical simulation via CFD software is provided for this interdisciplinary research.
In order to achieve the objectives, the process is divided into two parts, experimental measurements and numerical simulation. For experimental measurements, apparatus similar to Figure 2 should be used as a container for zebra fish, the tank is separated by a perforated wall into two compartments, according to the specific experiment requirements, the perforated wall can be removed. Webcam is used to record the swimming behavior of zebra fish.
Depending on the age of zebra fish, the apparatus can be different as well. For example, for zebra fish larvae or embryos, petri dish is used as the container. Once the video is recorded, a custom-made Matlab algorithm will process the video to get the useful parameters such as average velocity, tail beat frequency and amplitude. Typical shape of zebra fish will be captured and kept for future works. Once the pre-processing of data is finished, we will move onto the numerical simulation part.
As for the numerical simulation, CFD is a commonly used tool. We use OpenFOAM, which is an open source CFD software to simulate the motion of zebra fish. Firstly, we assume that the zebra fish can be divided into several parts like head, trunk and tail; each part is seen as rigid body and is connected to form the whole body. In order to simulate the swimming behavior accurately, typical shapes of zebra fish having captured can be used to build the model, some parameters like velocity, tail beat frequency and amplitude can be seen as input parameter to simulate movements of specific parts of zebra fish. Once the movement for each rigid body is achieved, influences among each part can be studied via post-processing of the movements like forces, moments, vortices, etc. by comparing the differences between the normal zebra fish and zebra fish with muscle dystrophy at different ages, we can see the influences of muscle dystrophy on skeletal muscle and the corresponding swimming behavior in the life span. In addition to the application in developing new medicine for muscle dystrophy, once the OpenFOAM program is developed, the program can be applied in the biomimetic field to test the maximum propulsive efficiency for the model with different combination of movements.
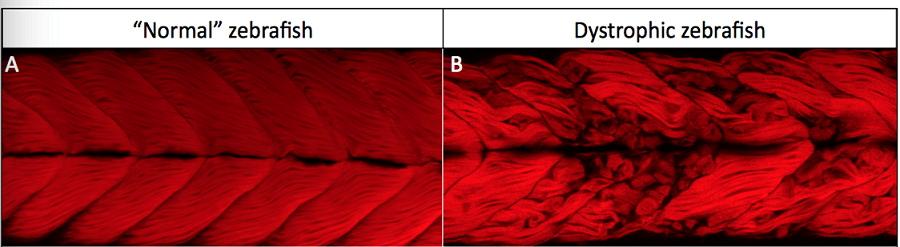
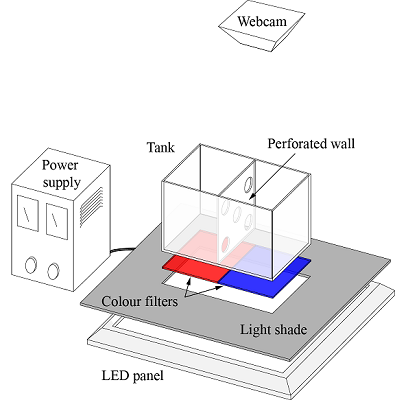
Numerical Simulations on Bio-inspired Swimming Robots
By Ruoxin Li, Professor Qing Xiao
This research is a numerical simulation, based on the bio-inspired swimming robots: the Amphibot III. There are eight segments in the model, representing the fish backbone from the head to the tail. The desired shape motion is defined beforehand. Commercial software ANSYS Fluent 14.5 is used to carry out the calculation. To solve the motion problem for several different segments, the user defined function (UDF) in Fluent is used, which incorporates a multi-body dynamics algorithm. Two different groups of parameters are employed in order to imitate different fish locomotion. The vorticity contour is shown in both videos.
Further research on this problem, the anguilliform swimming is studied. By given analytical description, the width of fish body can be set to its length. The shapes of eight elements are changed in order to fit real anguilliform fish. As the lateral displacement of the mid-line is given, motions on the hinges can be curve fitted through dividing the fish length into 8 parts. Motions on all the joints are actuated. Animation is given as following. For the cases of passive joints, research will be carried out in the future.
Numerical Simulations on an Articulated Fish-Like System
By Jianxin Hu, Professor Qing Xiao
The research on fluid dynamics of biomimetic is a challenging problem due to the complex hydrodynamic mechanisms of real aquatic animals. Although there are existing investigations on the fish swimming mechanisms by establishing the numerical and experimental models with typical kinematic motions, the understandings are still limited on the fish body internal forces and response to external forces. Inspired by the previous work of Kanso (2005) and Eldredge (2008) on the propulsion study for an articulated multi-segment system, we successfully developed a numerical strategy for simulating the fish body consisting multi-segments based on a method of coupling the Computational Fluid Dyanmics (CFD) and Multi-Body Dynamics (MBD).
The studies on the propulsion and maneuvering abilities provide essential insight for the design of swimming robots and AUV, and the research results will obviously contribute on the methodologies improvements and biomimetic understanding of internal and external forces response mechanisms.
With the use of this multi-element system to emulate fish body rather than using one specified travelling wave along body (Figure 1), the replication of the multiple degrees of freedom of real fish body along with the body internal inertial forces becomes feasible. The preliminary validation result in Figure 2 presents the feasibility and reliability of the in house code. The research interest is further developed on an integrated analytical-simulation frame work to model multi-segments robots, Amphibot III (Figure 3), by collaborating with the robotic team in Institut de Recherche en Communication et Cybernétique de Nantes (IRCCyN). The task is to incorporating the dynamic model in Amphibot III into CFD codes, and the expected results can be seen as: a) visible flow field around the robot; b) detailed comparison and validation between CFD results and LAEBT analytical results.




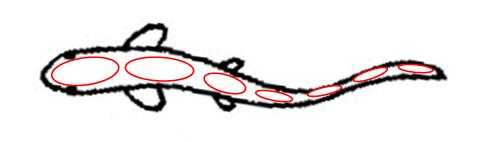
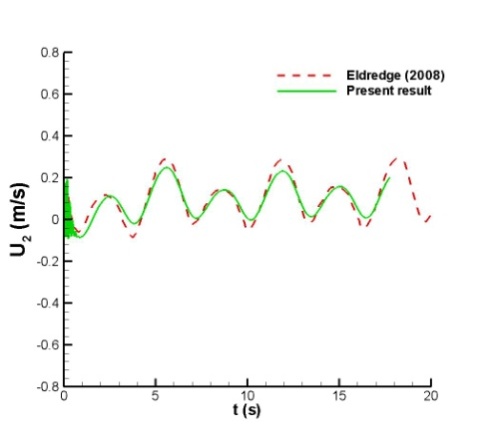
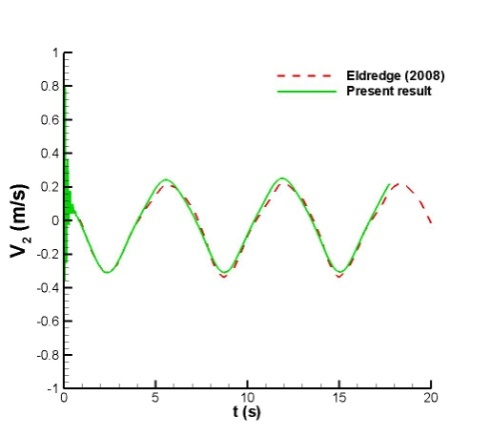
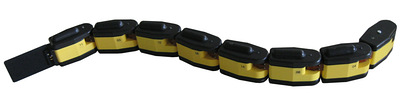
Response of a flexible filament in a flowing soap film subject to a forced vibration
By Laibing Jia, Professor Qing Xiao
The interactions between flxible plates and flids are important physical phenomena. A flg in wind is one of the most simplifid and classical models for studying the problem. In this project, we investigated the response of a flg in flw with an externally forced vibration by using flxible fiaments and soap fim.
A model study has been carried out using flexible filament and a soap film tunnel. By applying an external forced vibration on the leading edge of the filament, we investigated the response of a flag in flow with an externally forced vibration by using flexible filaments and soap film. Experiments show that for a filament that is either in oscillation or stationary, the external forced vibration leads to its oscillation. A synchronization phenomenon occurs in the experiments. A small perturbation leads to a large response of flapping amplitude in response. The insight provided here is helpful to the applications in the flow control, energy harvesting and bionic propulsion areas.

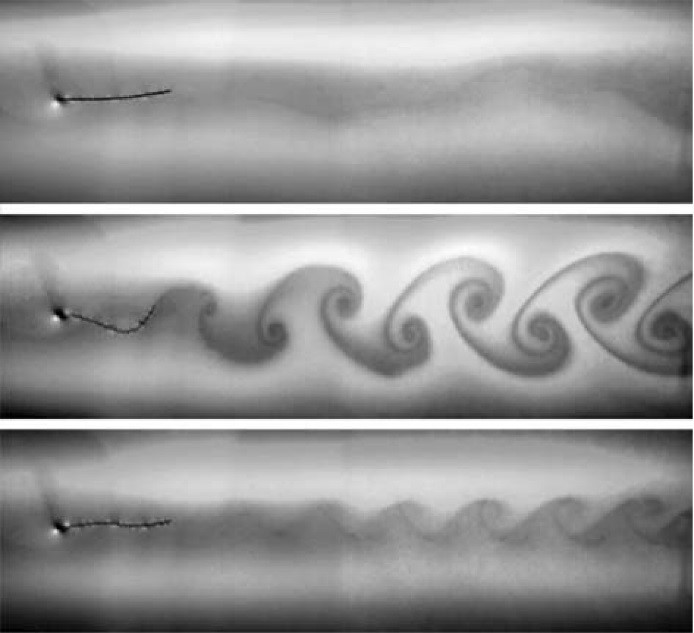
Three Dimensional Effects on the Translational Locomotion of a Passively Heaving Wing
By Jianxin Hu, Professor Qing Xiao
Inspired by the novel flapping-wing mechanisms in nature flyers of insects, birds and bats, we have carried out a numerical study systematically investigating a three-dimensional flapping rigid wing with the passively actuated lateral and rotational motion. Distinguishing from the limited existing studies, our work performs a systematic examination on the effects of wing aspect ratio, inertia, torsional stiffness and pivot point on the dynamics response of a low aspect ratio rectangular wing under an initial zero speed flow field condition.
Marine animal wings and foils perform remarkable swimming abilities by creating high thrust speed and flexible maneuverability. This research on the investigation of propulsion mechanisms of flapping wings is both of interest to the MAV community and of importance to comparative morphologists when they are considering how physics constrains biological design. The assessment tool can also be used to predict the performance of various bio-inspired marine applications.
The simulation results show that the symmetry break-down of the flapping wing results in a forward/backward motion with a rotational pitching, as shown in Figure 1. Depending on various kinematic and dynamic system parameters, the lateral induced velocity shows a number of different hydrodynamic performances, for example, wing under faster plunging frequencies develop a wake structure with a smaller vortex angle (Figure 2), which can slow down the energy dissipation, therefore the wing is able achieve a faster forward speed.
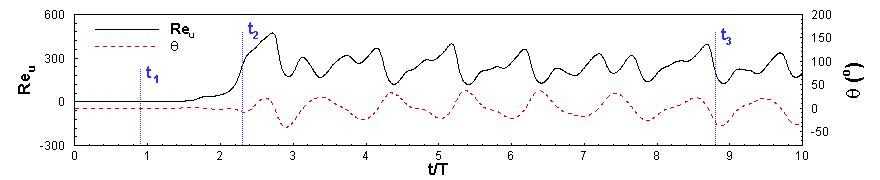
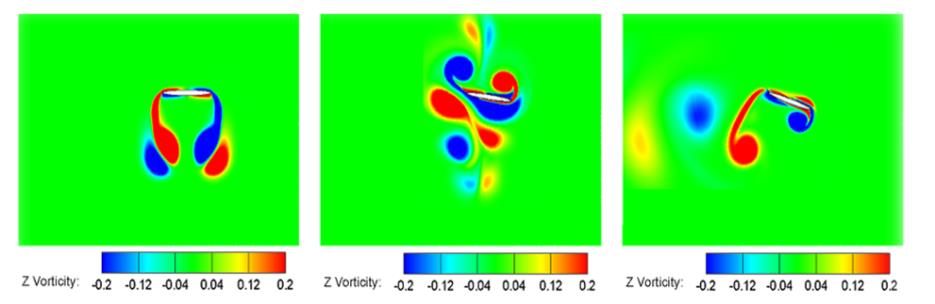
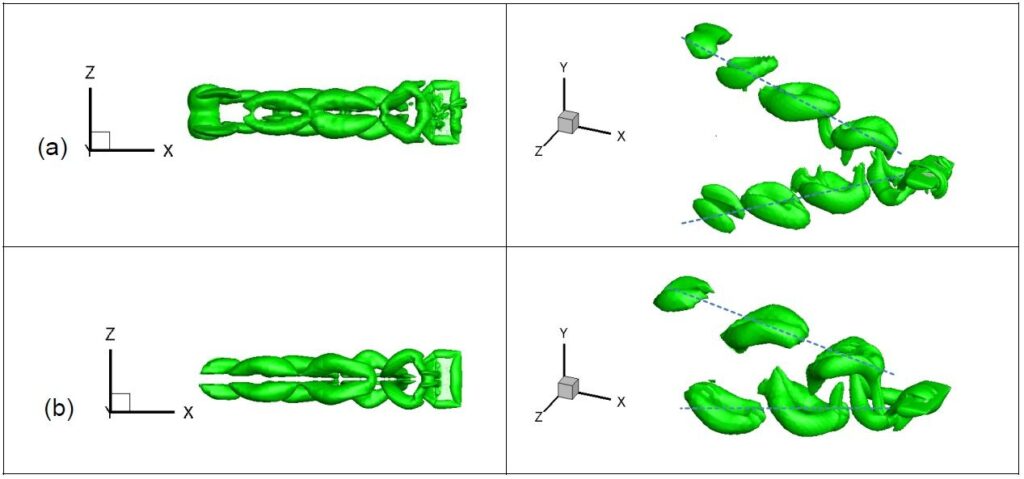
Parametric Study on a Cylinder Drag Reduction Using Downstream Undulating Foil
By Wendi Liu, Professor Qing Xiao
This study is a flow system with a D-sectional cylinder combined with an undulating NACA0012 foil in the wake of cylinder. Cylinder drag force could be considerably reduced if the foil is properly placed in the cylinder wake. In this study, a detailed parametric study on this coupled cylinder-undulating-foil system is carried out by numerical simulation. Particular interest is focused on how Reynolds number, relative size of foil and cylinder, foil undulating frequency, wavelength and gap between cylinder and foil affect the cylinder drag, lift force as well as foil thrust. For a range of flow and geometry parameters studied here, our results show that the maximum cylinder drag and lift coefficient can reduce as 57.4% and 63.3% relative to the cylinder without undulating foil, respectively. Foil thrust coefficient increases to 4 times as compared to single foil. Distinguished from conventional cylinder vortex control method, coupled cylinder-undulating foil system provides some new insights on the vortex control and suppression mechanism.
This numerical study examines the potential of cylinder wake vortex control using a downstream undulating foil. It distinguishes itself considerably from our recent research, which focuses on the propulsion performance enhancement of undulating foil by extracting energy from the upstream cylinder wake. The present investigation covers a much wider range of kinematic and geometric parameters of this coupled cylinder�Cfoil system, such as the foil undulating frequency Stf, wavelength (λf) and the gap ratio (L/c) and cylinder-foil relative size (D/c). It aims for obtaining an optimal parametric range to achieve a significant cylinder drag reduction, which is impossible to be accomplished by our earlier study on the limited parameters. Our results show that the proper placement of foil, with its chord length equal to the upstream cylinder diameter, can result in a suppression of cylinder vortex shedding and an improvement in downstream foil propulsion. The detailed flow field analysis reveals that, such benefit is strongly linked to the interaction between the cylinder wake vortex and the reversed Karman vortex street, which is shedding continuously at the foil leading edge associated with foil undulation locomotion. This new finding undetected by preceding investigations prompts further study in the near future.
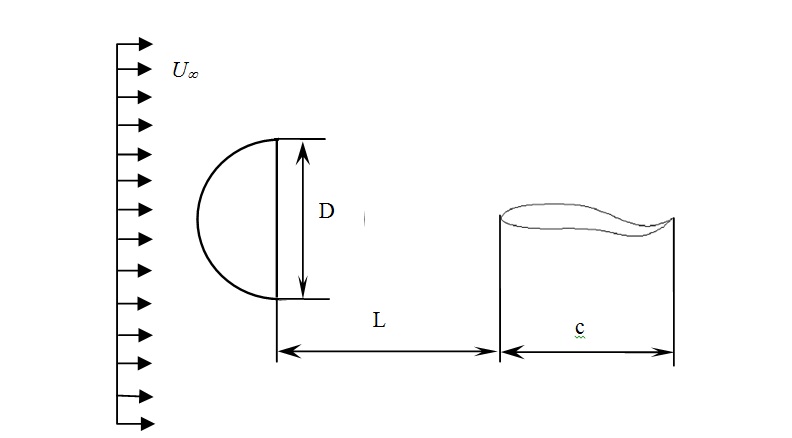
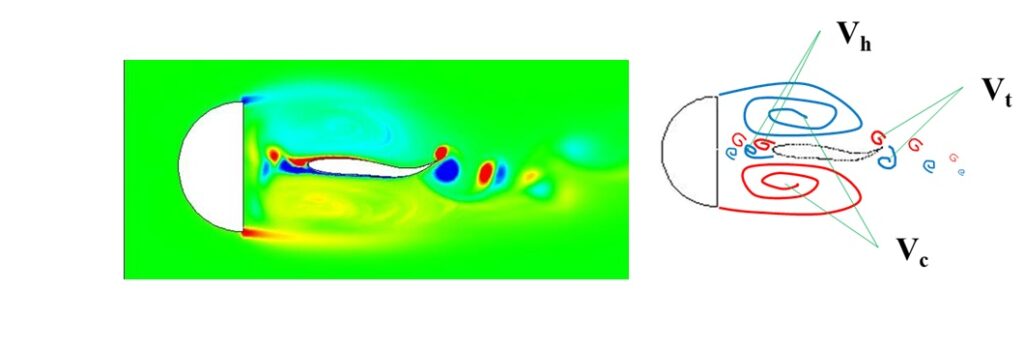